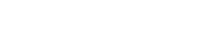
How Vacuum Decay Would Destroy The Universe
Season 7 Episode 27 | 13m 1sVideo has Closed Captions
Of all the possible ends of the universe, vacuum decay would be the most thorough.
The universe is going to end. But of all the possible ends of the universe vacuum decay would have to be the most thorough - because it could totally rewrite the laws of physics. Today I hope to help you understand exactly how terrified you should be.
Problems with Closed Captions? Closed Captioning Feedback
Problems with Closed Captions? Closed Captioning Feedback
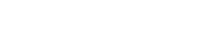
How Vacuum Decay Would Destroy The Universe
Season 7 Episode 27 | 13m 1sVideo has Closed Captions
The universe is going to end. But of all the possible ends of the universe vacuum decay would have to be the most thorough - because it could totally rewrite the laws of physics. Today I hope to help you understand exactly how terrified you should be.
Problems with Closed Captions? Closed Captioning Feedback
How to Watch PBS Space Time
PBS Space Time is available to stream on pbs.org and the free PBS App, available on iPhone, Apple TV, Android TV, Android smartphones, Amazon Fire TV, Amazon Fire Tablet, Roku, Samsung Smart TV, and Vizio.
Providing Support for PBS.org
Learn Moreabout PBS online sponsorshipThe universe is going to end.
But of all the possible ends of the universe vacuum decay would have to be the most thorough - because it could totally rewrite the laws of physics.
Today I hope to help you understand exactly how terrified you should be.
It seems pretty lucky that the universe is how it is.
Its just the right size, has just the right expansion rate, and has just the right particle properties to allow stars and planets and people to exist.
The habitability of our universe is largely defined by the properties of the quantum fields that pervade all space.
The quantum fields give rise to the particles that make up all matter and all forces - and if those quantum fields were a bit different, none of the familiar structures from atoms to galaxies - would be possible.
In fact, for most possible configurations of the quantum fields, no structures would exist at all.
Fortunately things are they way they are … although don’t get too cosy - there’s at least one mechanism that could rewrite the laws of physics across the universe.
That mechanism is vacuum decay, and some physicists think it’s inevitable.
First to give you a crude picture - vacuum decay looks like a bubble of annihilation that expands at the speed of light, rewriting the nature of the quantum fields as it passes.
To understand whether and when this might happen, we first need to understand the quantum fields that it threatens.
For that let’s try an analogy.
Think of all space as being sort of springy at every point.
As a simplistic example, imagine a rubber ring at each point.
If you compress the ring in one direction it bounces back and begins to oscillate around its equilibrium shape.
But each ring is also connected to its neighboring rings, and so it can transfer its oscillations, causing a wave to propagate through space.
And there are other ways for the rings to oscillate - it has different vibrational modes.
For example it could twist back and forth on different axes, or deform or more complex ways.
We can think of each quantum field as a set of these modes of oscillation.
And each quantum field - each type of oscillation - has a corresponding particle - that’s the oscillation itself.
Just like the deformed ring, a quantum field wants to return to its equilibrium position.
That position is the field value where the energy is minimized.
Physicists like to represent this by plotting the energy in the quantum field versus field value.
It takes more energy to get further away from the minimum - the equilibrium point, just like it takes more energy if you want to deform the rubber ring.
Another nice physical analogy is a ball rolling in a dip.
The ball will roll up and down, oscillating around the equilibrium, and the height it reaches depends on how much energy it has.
For most quantum fields, the minimum energy is where the field value is zero.
For example, for an electromagnetic wave - a photon - the electric and magnetic fields rise and fall between positive and negative values, but the average field value is zero.
And after the photon passes the electromagnetic field goes back to zero.
But there’s one quantum field that breaks these rules.
That’s the Higgs field.
The minimum energy state of the Higgs field is not where the field strength is zero.
Instead, the Higgs field likes to settle into an equilibrium value where it has a real, positive value.
This means that the entire universe is filled with this soup of Higgsiness.
Most elementary particles that have mass gain their mass due to interactions with this ubiquitous field.
I go into all the detail of the mass-granting power of the Higgs mechanism in a previous video.
For today, all you need to know is that there's this one quantum field that has a minimum energy value where the field strength is non-zero - and that this also means that the energy carried by the Higgs field is non-zero, even at this minimum.
And that's where the trouble starts.
The Higgs field may have yet another weird property.
It may have more than one possible minimum value.
This would look like multiple dips in our graph of energy versus field strength.
A quantum field with multiple minima like this will tend to find its way into one of these dips and get stuck there.
Just like a ball rolling on an undulating surface.
In order to move between dips, the ball, or the quantum field, needs to gain enough energy to overcome this barrier between them.
That could happen, say, in an extreme energy environment like the big bang or near a black hole or in a sufficiently large particle accelerator.
But there’s another to make this jump.
The Heisenberg uncertainty principle tells us that there’s a fundamental uncertainty in - well, pretty much everything, but certainly in the value of a quantum field.
This results in fluctuations in the field strength that can cause it to spontaneously shift, and perhaps find itself stuck in an adjacent dip.
We call this quantum tunneling.
So what does this mean for the Higgs field?
Well some theorists do believe that the Higgs field has at least two minima, and those minima should have different energy values.
There’s a true minimum - the true lowest energy, or what we call the true vacuum.
This is where the field would prefer to spend its time given the choice.
And then there’s another local minimum with a higher energy - what we call that a false vacuum.
The false vacuum is a so-called metastable state - it seems stable as long as the field doesn’t learn about the more stable, lower energy state.
Somewhat worryingly, we don’t know which of these minima our universe’s Higgs field is in right now.
Let’s think about the potentially catastrophic consequences of this.
Firstly, if the Higgs field is in the true minimum then no big deal.
Even if the field at one location of space tunneled or was excited into the false minimum, it would quickly find its way back to the true minimum for reasons I’ll get to.
But what if the entire universe is in the false vacuum?
Then a tunneling event would be a little more dramatic.
Imagine a universe filled with the Higgs field in a false vacuum.
At a single point in space, a quantum tunneling event drops the field into the true vacuum.
This creates a tiny bubble that is at an energetically favorable state compared to the surroundings.
The interior of the bubble immediately tries to drag the surrounding Higgs field down with it.
This happens because quantum fields are connected and tug at their adjacent points across space, which is why their oscillations propagate as particles.
Now this isn’t necessarily an instant disaster.
The interior of the bubble wants to expand, but the shell of the bubble is actually in an energetically unfavorable state - it’s in this unpleasant transitional state between the two minima of the Higgs field.
In fact, the bubble wall experiences a sort of surface tension that tries to collapse the bubble again.
Things get awkward if the initial bubble exceeds a certain size.
The bigger the bubble, the more its interior wants to expand into its surroundings.
But you also have more bubble surface, so more surface tension to resist that expansion.
But the interior of the bubble increases with the cube of the radius, while the surface increases only with radius squared.
That means that above a certain size, the hunger of the bubble interior dominates.
The bubble grows, and that growth very quickly approaches the speed of light.
Once it gets going, the bubble is unstoppable, and will drag the Higgs field through the entire universe down into the true vacuum.
This is vacuum decay.
It’s a phase transition of the quantum fields.
In fact it has a lot of similarities with the sort of phase transitions that you get in matter.
For example, when water boils - it undergoes a phase transition to water vapor - that phase transition also starts in small bubbles that grow into its surroundings.
The formation of these phase-transition bubbles in water or in a quantum field is called bubble nucleation.
OK, let’s review the horrors that would result from vacuum decay.
First up, everything gets fried.
The energy released in the decay of the Higgs field fills the expanding bubble with a hot soup of energetic particles.
It’s similar to how the energy held in the latent heat of boiling water is released into the kinetic energy of gas molecules.
But that’s not the worst of it.
As I mentioned, the Higgs field gives elementary particles their masses.
Those masses depend on the energy in the field - the so-called vacuum expectation value.
Drop the energy in the field and you reduce the masses of the elementary particles.
The ability for stars to form and undergo nuclear fusion, and the ability for chemistry to work as it does, depend sensitively on the properties of the elementary particles.
Life and structure could not exist as we know it, and may not exist at all.
I should also add that there are other possible types of vacuum decay beyond Higgs field decay.
For example, there may be weird fields within string theory that exist in false vacuum states.
The decay of those could rewrite the laws of physics in far more drastic ways.
This all sounds moderately bad.
But can this actually happen?
First let’s ask whether we really are living in one of these nasty false vacuums - then we’ll talk about whether that vacuum might decay.
Assuming the Higgs field really does have multiple minima, the question becomes which minimum are we in?
The true one or a false one?
It turns out that the theorists aren’t sure.
We can determine the shape of the Higgs field with precise measurements of the particles that gain their mass from the Higgs.
The most important are the Higgs particle itself as well as the top quark, which is the most massive elementary particle.
And our measurements of these masses tell us that … we are probably in the false vacuum - but we’re actually very close to the boundary.
We MIGHT be in the true vacuum - which would be good, but it’s a bit more likely that we’re in the bad, false one.
Further study of the Higgs particle and top quark should help us figure this out.
OK, so the next question is could the vacuum really decay?
As long as decay is possible, it’s inevitable.
At any instant in time there’s a tiny but real probability that a patch of space will tunnel between the false and true vacuums.
Wait long enough and it WILL happen.
Happily, there’s only a very small probability that a bubble of true vacuum will randomly form that’s large enough to actually continue growing.
Physicists have not converged on a single timescale - they vary from roughly the current age of the universe to 10^hundreds times the age of the universe for a single such bubble to be likely to appear somewhere in our observable universe.
So, somewhere between vaguely unlikely and staggeringly unlikely.
Before you get too comfortable, remember there’s another way to hop between energy minima.
If enough energy can be pumped into a patch of space, the Higgs field can hop between minima without tunneling.
This would be like dropping specks of dust into superheated water - dust provides sites for bubble nucleation, and the entire body of water rapidly evaporating.
Now, there was some fear that our giant particle colliders like the LHC might nucleate a vacuum decay bubble.
But that was unfounded.
After all, Earth is constantly being bombarded by cosmic rays of much, much higher energy than the LHC can produce, and no annihilation just yet.
Overall, it’s very unlikely that a vacuum decay bubble will reach us in the lifespan of our species, let alone your own lifetime.
Of course, in an infinitely or sufficiently large universe then vacuum decay has definitely started somewhere.
But as long as we’re far enough away we’re safe.
If the vacuum decay starts beyond several billion light years, the accelerating expansion of the universe will throw us away from it faster than the bubble of annihilation can grow, even though that growth is at nearly the speed of light.
And this leads to one more comforting fact.
If the vacuum does decay inside our cosmic horizon we’ll never see it coming.
Because no light can outpace the expanding bubble to warn us of its approach.
One moment you might be watching your favorite YouTube show, the next … well, there would be no next.
Who knows - perhaps the bubble edge has already swallowed your computer screen a nanosecond ago.
… I guess not.
Good.
So, just for now let’s enjoy whatever time we have left - perhaps mere billions of years - before vacuum decay swallows this fragile and metastable space time.
Support for PBS provided by: